Why do some memories last a lifetime while others fade away? A groundbreaking new study sheds light on this mystery by uncovering hidden patterns of brain activity that support long-term memory. Using a framework inspired by thermodynamics, scientists have developed a novel approach to understanding how different brain regions work together to shape cognition.
The second law of thermodynamics tell us that all complex living systems operate far from equilibrium, leading to the emergence of the ‘arrow of time’. This results in their dynamic activity being time-irreversible. The human brain is no exception, and is an example of a superbly complex system with many interactions between a wide array of specialised brain areas. Inferring which brain regions are engaging in a significant interaction that is facilitating cognition remains an important challenge in neuroscience. Inspired by the study of thermodynamics, the time-irreversibility of neural activity can be used to identify key groups of regions whose interactions underly cognition at a number of scales.
In a study published in the journal PNAS, the ‘DiMViGI’ framework was presented for measuring the irreversibility of neural recordings across different levels of interaction. Human participants engaged in a long-term memory task that tested their ability to identify variations of a short musical piece. When they were able to correctly identify the piece, their brain dynamics, recorded with magnetoencephalography, were analysed using a graph-theoretic method, that quantified the irreversibility of regional interactions. The striking results show that the thermodynamics-inspired method was able to differentiate between significant and insignificant interactions between groups of brain regions.
In an interdisciplinary effort, bringing together mathematicians and neuroscientists from the Universities of Oxford, Aarhus, and Pompeu Fabra, the researchers offer a new perspective on the analysis of brain network dynamics, with particular focus on long-term recognition. The findings showed that causal interactions, such as those between sensory and processing regions, were significantly more irreversible than less causal interactions, such as those between a pair of sensory regions.
For simplicity, interactions in complex systems are often analysed at the pairwise level. Nevertheless, many systems are actually comprise ‘higher-order’ interactions that include ensembles of three or more elements simultaneously. Using their novel framework, the researchers were able to identify these higher levels of interaction directly from the neural activity. More specifically, the findings show that medial regions could play a crucial role in orchestrating the long-term recognition by facilitating communication between hemispheres in a ‘higher-order’ fashion.
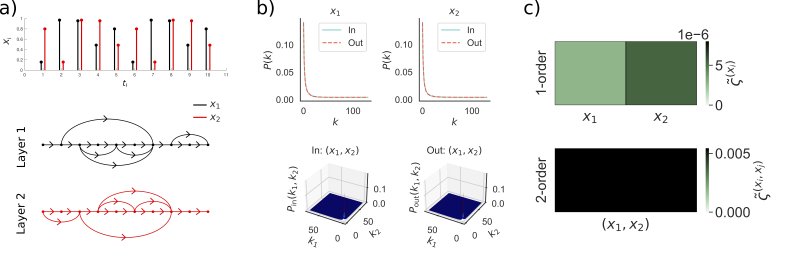
Image above: the DiMViGI framework. a) A multivariate neural recording is first mapped into a multilayer graph called a ‘visibility graph’. b) We then calculate the multivariate marginal in- and out- degree distributions across all levels of the system. c) By computing the divergence between a specific in- and out-degree marginal, we obtain a measure of the irreversibility of a particular interaction.
“Statistical physics and neuroscience have often proved to have a synergistic relationship, with each inspiring developments in the other. Our study builds on the developing area of analysing neural dynamics from the perspective of nonequilibrium thermodynamics. The results confirm that the language and tools of statistical physics, in this case irreversibility, can yield novel and exciting insights into the structure of interactions in human brain dynamics. Using a cutting-edge dataset that focuses on neural dynamics during long-term memory, we are able to illustrate the unique perspective that our framework can bring,” said Ramón Nartallo-Kaluarachchi, a DPhil candidate from the Mathematical Institute at the University of Oxford.
“This study provides unique insights into multivariate interactions between brain regions, systematically identifying the specific configurations of sets of brain region where the strongest interactions emerge. These findings enhance our understanding of functional brain communication and offer a novel tool for advancing cognitive research,” said Professor Leonardo Bonetti, from the Centre for Music in the Brain at Aarhus University.
By leveraging this novel framework, this study breaks new ground by identifying those higher-order relationships at the core of auditory recognition.
The team working on the research comprises Ramón Nartallo-Kaluarachchi (author of this piece), Leonardo Bonetti, Gemma Fernández-Rubio, Peter Vuust, Gustavo Deco, Morten L. Kringelbach, Renaud Lambiotte and Alain Goriely.