Mathematical Modelling of Silicon Furnaces
- Researcher: Ben Sloman
- Academic Supervisors: Colin Please and Robert Van Gorder
- Industrial Supervisors: Aasgeir Valderhaug and Rolf Birkeland
Background
How can solar panels become cheaper? Part of the cost is in the production of silicon, which is manufactured in electrode-heated furnaces through a reaction between carbon and naturally occurring quartz rock (see Figure 1, reproduced from The Si Process Drawings, by Thorsteinn Hannesson). Making these furnaces more efficient could lead to a reduction in the financial cost of silicon and everything made from it, including computer chips, textiles, and solar panels. Greater efficiency also means reduced pollution into the air.
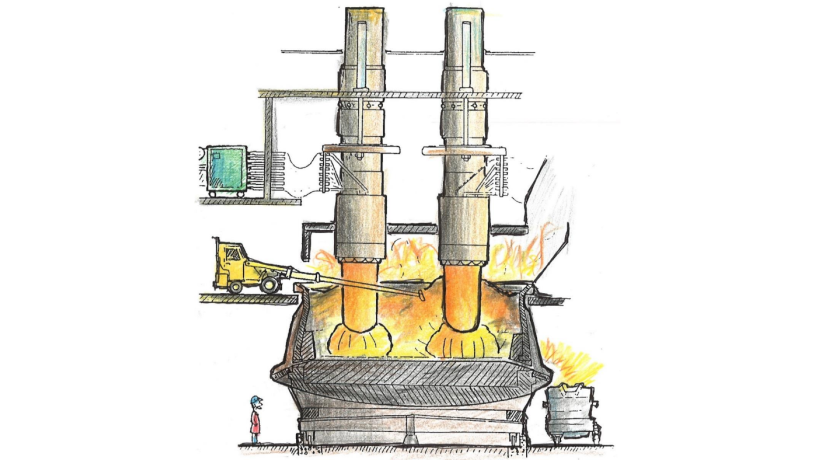
Figure 1: A sketch of a silicon furnace. Reproduced from The Si Process Drawings, by Thorsteinn Hannesson.
One problem in silicon production is the formation of a solid crust, which clogs up the furnace and prevents the raw materials from falling down the furnace to the hot region, where the necessary chemical reactions occur. Due to the high temperatures involved (around 2000 kelvin) it is difficult to observe how this clogging happens, so Elkem have carried out experiments on `pilot furnaces.’ These experiments comprise of graphite cylinders filled with the industrial raw material, and heated by an induction heater.
In this project we seek to understand the role of chemical, thermal, material transport, and mechanical effects inside silicon furnaces. The modelling and analysis are focussed on the pilot furnace experiments, where electrical effects do not need to be considered and the geometry is simplified.
Outcomes
Since the dominant effects in the pilot furnace are unidimensional, we have developed a 1-D model of coupled PDEs which captures the evolution of chemical concentrations, temperature, and gas flow, with the spatial variable being height in the furnace [1]. Numerical simulations of the model compare well with the experiments (see Figure 2), and demonstrate that the position of crust formation is largely driven by temperature, with the location moving upwards as the furnace becomes hotter. The furnace operators can change the type of raw materials used in the process and the energy input into the electrodes. Simulations of the model show that using more reactive carbon particles (for example charcoal) reduces the amount of silicon monoxide gas escaping from the furnaces, allowing more silicon to be produced from the quartz, and also reducing the build up of the furnace crust.
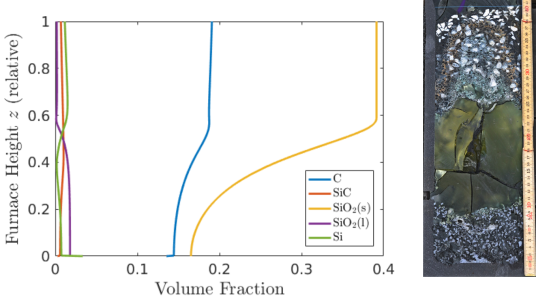
Figure 2: Left: Result of a numerical simulation showing the volume fraction of different solid and liquid chemicals found in the furnace. Right: The pilot furnace experiment – the green area is epoxy resin, injected to fill a gas cavity.
We have performed an asymptotic analysis of this model, in order to determine the dominant chemical and thermal behaviour of the system [2]. This was done by exploiting the relative rates of different chemical reactions, and that gas convects faster than any chemical timescale. The original model consists of nine equations for nine unknowns, but through systematic reductions we obtain a simpler model of two coupled equations for the temperature and the concentration of solid quartz. These equations account for diffusion, an endothermic reaction, and the external heating input to the system, and capture the same qualitative behaviour as the more complex model (see Figure 3).
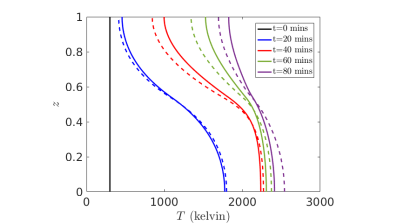
Figure 3: Results of numerical simulations for the simplified model (dotted lines) with the full model (solid lines), for temperature in the pilot furnace.
Through considering a 1-D chemical and thermal model we have been able to determine the location of the crust formation, but not understand how the crust can support the weight of the solid material above a gas cavity. To address this, we have also developed a thermo-mechanical model, where the crust is represented by a viscous fluid with a thermally-dependent viscosity, and is heated by radiation from below. Depending on parameter values, the system can tend towards a stable steady state, where the crust is static at a given height in the furnace and acts as a blockage. Alternatively, for other parameter values, the furnace charge can undergo a desired free fall, as happens in the ferro-silicon process.
In the furnace the reaction between solid carbon and gaseous silicon monoxide is crucial for silicon production. Reactions between gases and solid particles are commonly modelled using a shrinking core framework, where a sharp interface between an inner unreacted core and an outer product shell moves inwards until the reaction is complete. We develop a homogenised model for a large porous solid particle made up of many small grains, by assuming a shrinking core model represents behaviour at the microscale level and exploiting the small ratio of granular to particle length scales [3]. These macroscale equations allow for a diffuse reaction front, as well as a sharp interface between reacted and unreacted solid material. As such, this is a better representation of reaction behaviour than the simple shrinking core model.
Publications
[1] B. M. Sloman, C. P. Please, R. A. Van Gorder, A. M. Valderhaug, R. G. Birkeland, and H. Wegge. A heat and mass transfer model of a silicon pilot furnace. Metallurgical and Materials Transactions B. 48(5), (2017), 2664-2676.
[2] B. M. Sloman, C. P. Please, and R. A. Van Gorder. Asymptotic analysis of a silicon furnace model. SIAM Journal on Applied Mathematics, 78(2), (2018), 1174-1205.
[3] B. M. Sloman, C. P. Please, and R. A. Van Gorder. Homogenisation of a shrinking core model for gas-solid reactions in granular particles. Submitted on 4/12/2017.